Synthesis and electrochemical characterization of silicon clathrates as anode materials for lithium ion batteries
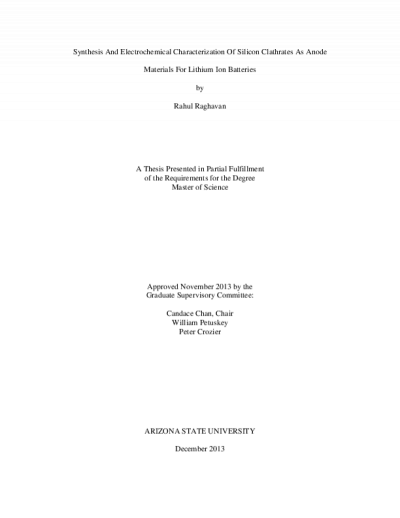
Description
Novel materials for Li-ion batteries is one of the principle thrust areas for current research in energy storage, more so than most, considering its widespread use in portable electronic gadgets and plug-in electric and hybrid cars. One of the major limiting factors in a Li-ion battery's energy density is the low specific capacities of the active materials in the electrodes. In the search for high-performance anode materials for Li-ion batteries, many alternatives to carbonaceous materials have been studied. Both cubic and amorphous silicon can reversibly alloy with lithium and have a theoretical capacity of 3500 mAh/g, making silicon a potential high density anode material. However, a large volume expansion of 300% occurs due to changes in the structure during lithium insertion, often leading to pulverization of the silicon. To this end, a class of silicon based cage compounds called clathrates are studied for electrochemical reactivity with lithium. Silicon-clathrates consist of silicon covalently bonded in cage structures comprised of face sharing Si20, Si24 and/or Si28 clusters with guest ions occupying the interstitial positions in the polyhedra. Prior to this, silicon clathrates have been studied primarily for their superconducting and thermoelectric properties. In this work, the synthesis and electrochemical characterization of two categories of silicon clathrates - Type-I silicon clathrate with aluminum framework substitution and barium guest ions (Ba8AlxSi46-x) and Type-II silicon clathrate with sodium guest ions (Nax Si136), are explored. The Type-I clathrate, Ba8AlxSi46-x consists of an open framework of aluminium and silicon, with barium (guest) atoms occupying the interstitial positions. X-ray diffraction studies have shown that a crystalline phase of clathrate is obtained from synthesis, which is powdered to a fine particle size to be used as the anode material in a Li-ion battery. Electrochemical measurements of these type of clathrates have shown that capacities comparable to graphite can be obtained for up to 10 cycles and lower capacities can be obtained for up to 20 cycles. Unlike bulk silicon, the clathrate structure does not undergo excessive volume change upon lithium intercalation, and therefore, the crystal structure is morphologically stable over many cycles. X-ray diffraction of the clathrate after cycling showed that crystallinity is intact, indicating that the clathrate does not collapse during reversible intercalation with lithium ions. Electrochemical potential spectroscopy obtained from the cycling data showed that there is an absence of formation of lithium-silicide, which is the product of lithium alloying with diamond cubic silicon. Type II silicon clathrate, NaxSi136, consists of silicon making up the framework structure and sodium (guest) atoms occupying the interstitial spaces. These clathrates showed very high capacities during their first intercalation cycle, in the range of 3,500 mAh/g, but then deteriorated during subsequent cycles. X-ray diffraction after one cycle showed the absence of clathrate phase and the presence of lithium-silicide, indicating the disintegration of clathrate structure. This could explain the silicon-like cycling behavior of Type II clathrates.
Date Created
The date the item was original created (prior to any relationship with the ASU Digital Repositories.)
2013
Agent
- Author (aut): Raghavan, Rahul
- Thesis advisor (ths): Chan, Candace K.
- Committee member: Crozier, Peter
- Committee member: Petuskey, William T
- Publisher (pbl): Arizona State University