An anticipatory-lifecycle approach towards increasing the environmental gains from photovoltaic systems through improved manufacturing and recycling
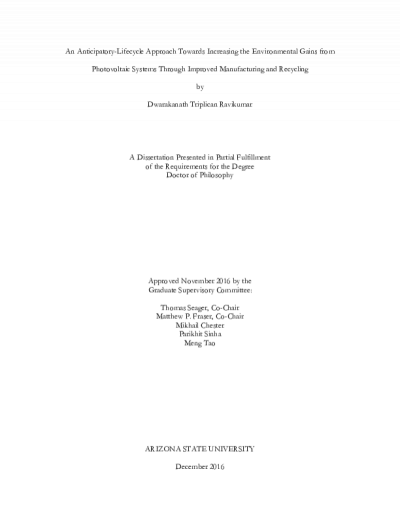
Description
Photovoltaics (PV) is an environmentally promising technology to meet climate goals and transition away from greenhouse-gas (GHG) intensive sources of electricity. The dominant approach to improve the environmental gains from PV is increasing the module efficiency and, thereby, the renewable electricity generated during use. While increasing the use-phase environmental benefits, this approach doesn’t address environmentally intensive PV manufacturing and recycling processes.
Lifecycle assessment (LCA), the preferred framework to identify and address environmental hotspots in PV manufacturing and recycling, doesn’t account for time-sensitive climate impact of PV manufacturing GHG emissions and underestimates the climate benefit of manufacturing improvements. Furthermore, LCA is inherently retrospective by relying on inventory data collected from commercial-scale processes that have matured over time and this approach cannot evaluate environmentally promising pilot-scale alternatives based on lab-scale data. Also, prospective-LCAs that rely on hotspot analysis to guide future environmental improvements, (1) don’t account for stake-holder inputs to guide environmental choices in a specific decision context, and (2) may fail in a comparative context where the mutual differences in the environmental impacts of the alternatives and not the environmental hotspots of a particular alternative determine the environmentally preferable alternative
This thesis addresses the aforementioned problematic aspects by (1)using the time-sensitive radiative-forcing metric to identify PV manufacturing improvements with the highest climate benefit, (2)identifying the environmental hotspots in the incumbent CdTe-PV recycling process, and (3)applying the anticipatory-LCA framework to identify the most environmentally favorable alternative to address the recycling hotspot and significant stakeholder inputs that can impact the choice of the preferred recycling alternative.
The results show that using low-carbon electricity is the most significant PV manufacturing improvement and is equivalent to increasing the mono-Si and multi-Si module efficiency from a baseline of 17% to 21.7% and 16% to 18.7%, respectively. The elimination of the ethylene-vinyl acetate encapsulant through mechanical and chemical processes is the most significant environmental hotspot for CdTe PV recycling. Thermal delamination is the most promising environmental alternative to address this hotspot. The most significant stake-holder input to influence the choice of the environmentally preferable recycling alternative is the weight assigned to the different environmental impact categories.
Lifecycle assessment (LCA), the preferred framework to identify and address environmental hotspots in PV manufacturing and recycling, doesn’t account for time-sensitive climate impact of PV manufacturing GHG emissions and underestimates the climate benefit of manufacturing improvements. Furthermore, LCA is inherently retrospective by relying on inventory data collected from commercial-scale processes that have matured over time and this approach cannot evaluate environmentally promising pilot-scale alternatives based on lab-scale data. Also, prospective-LCAs that rely on hotspot analysis to guide future environmental improvements, (1) don’t account for stake-holder inputs to guide environmental choices in a specific decision context, and (2) may fail in a comparative context where the mutual differences in the environmental impacts of the alternatives and not the environmental hotspots of a particular alternative determine the environmentally preferable alternative
This thesis addresses the aforementioned problematic aspects by (1)using the time-sensitive radiative-forcing metric to identify PV manufacturing improvements with the highest climate benefit, (2)identifying the environmental hotspots in the incumbent CdTe-PV recycling process, and (3)applying the anticipatory-LCA framework to identify the most environmentally favorable alternative to address the recycling hotspot and significant stakeholder inputs that can impact the choice of the preferred recycling alternative.
The results show that using low-carbon electricity is the most significant PV manufacturing improvement and is equivalent to increasing the mono-Si and multi-Si module efficiency from a baseline of 17% to 21.7% and 16% to 18.7%, respectively. The elimination of the ethylene-vinyl acetate encapsulant through mechanical and chemical processes is the most significant environmental hotspot for CdTe PV recycling. Thermal delamination is the most promising environmental alternative to address this hotspot. The most significant stake-holder input to influence the choice of the environmentally preferable recycling alternative is the weight assigned to the different environmental impact categories.
Date Created
The date the item was original created (prior to any relationship with the ASU Digital Repositories.)
2016
Agent
- Author (aut): Triplican Ravikumar, Dwarakanath
- Thesis advisor (ths): Seager, Thomas P
- Thesis advisor (ths): Fraser, Matthew P
- Committee member: Chester, Mikhail
- Committee member: Sinha, Parikhit
- Committee member: Tao, Meng
- Publisher (pbl): Arizona State University